Welding Information
Quote, Buy, Track!
We make it easy to get instant pricing and purchase your metal at the click of a button. Track your order progress, get notified when it ships, and follow your shipment online until it’s delivered. It’s that easy!
Latest articles,
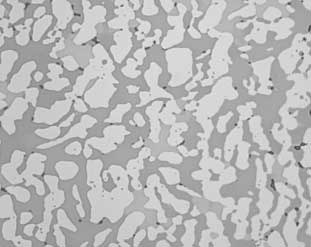
Quality of Microstructure of Duplex Stainless Steels
Duplex stainless steels typically have 50% of both austenite and ferrite in their microstructure. It is necessary to have good
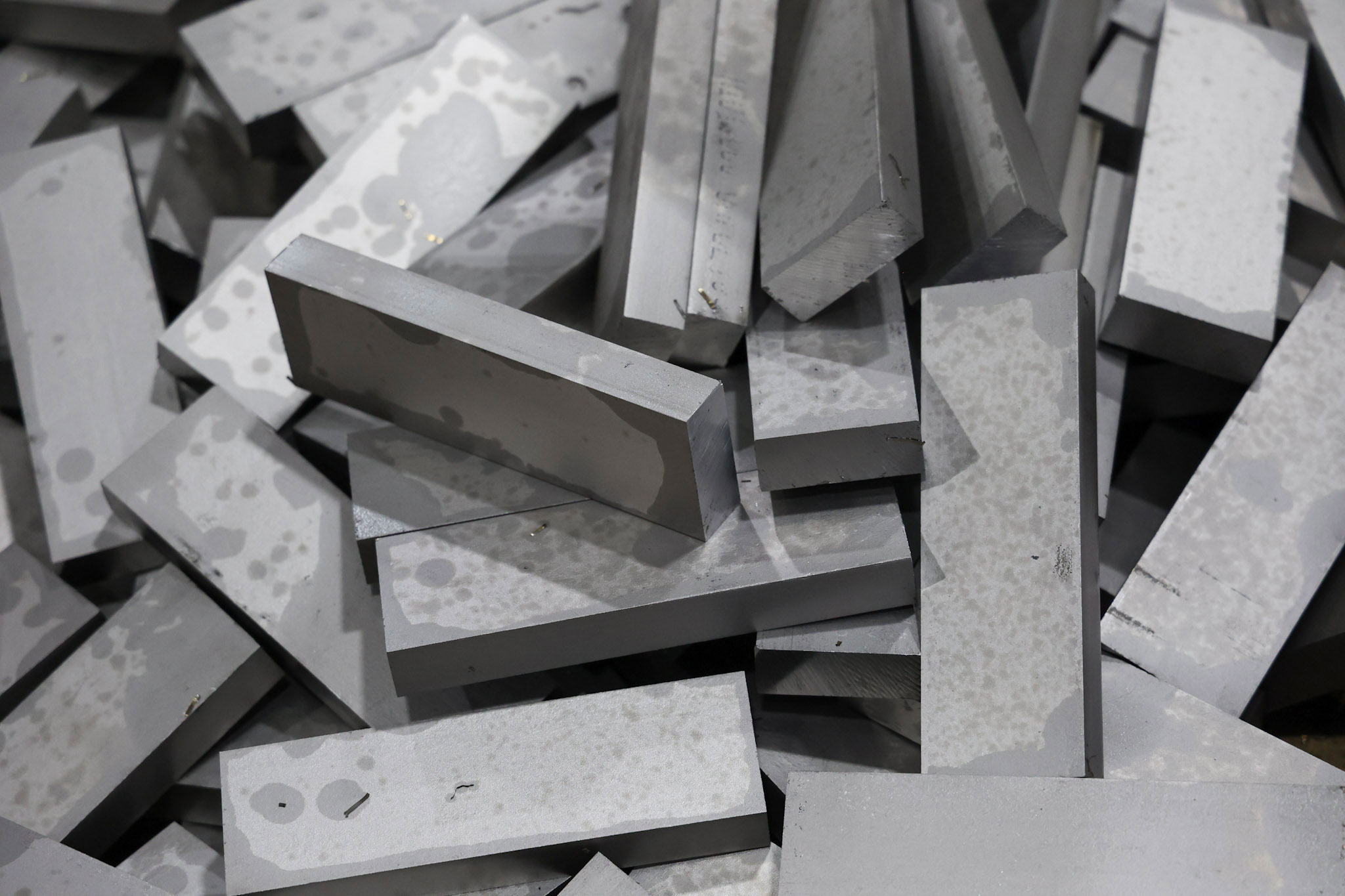
Flat Bar or Gauered Flat Bar from Plate
Discover the difference between flat bars and gauered flat bars cut from plates. Learn about their dimensional tolerances and the
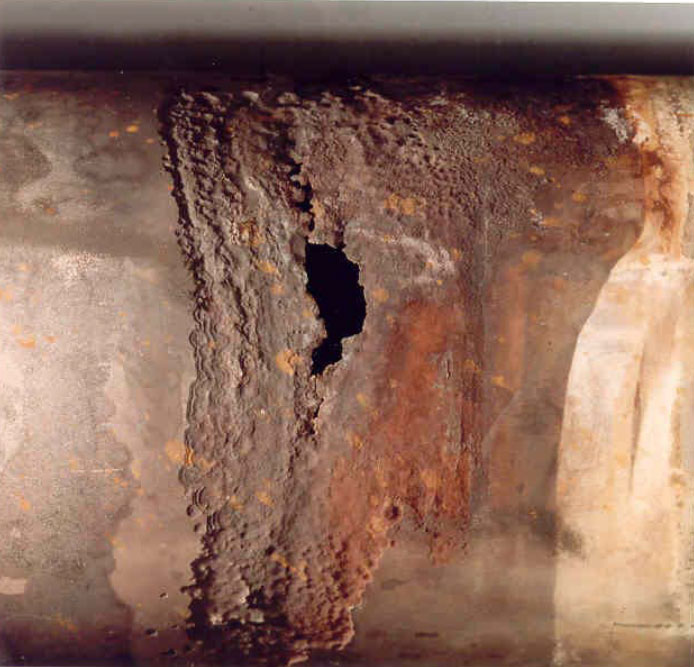
Metal Dusting
Metal Dusting is a hazard when using heat-treat carburizing furnaces and petrochemical equipment. Explore how high-chromium alloys thwart this destructive
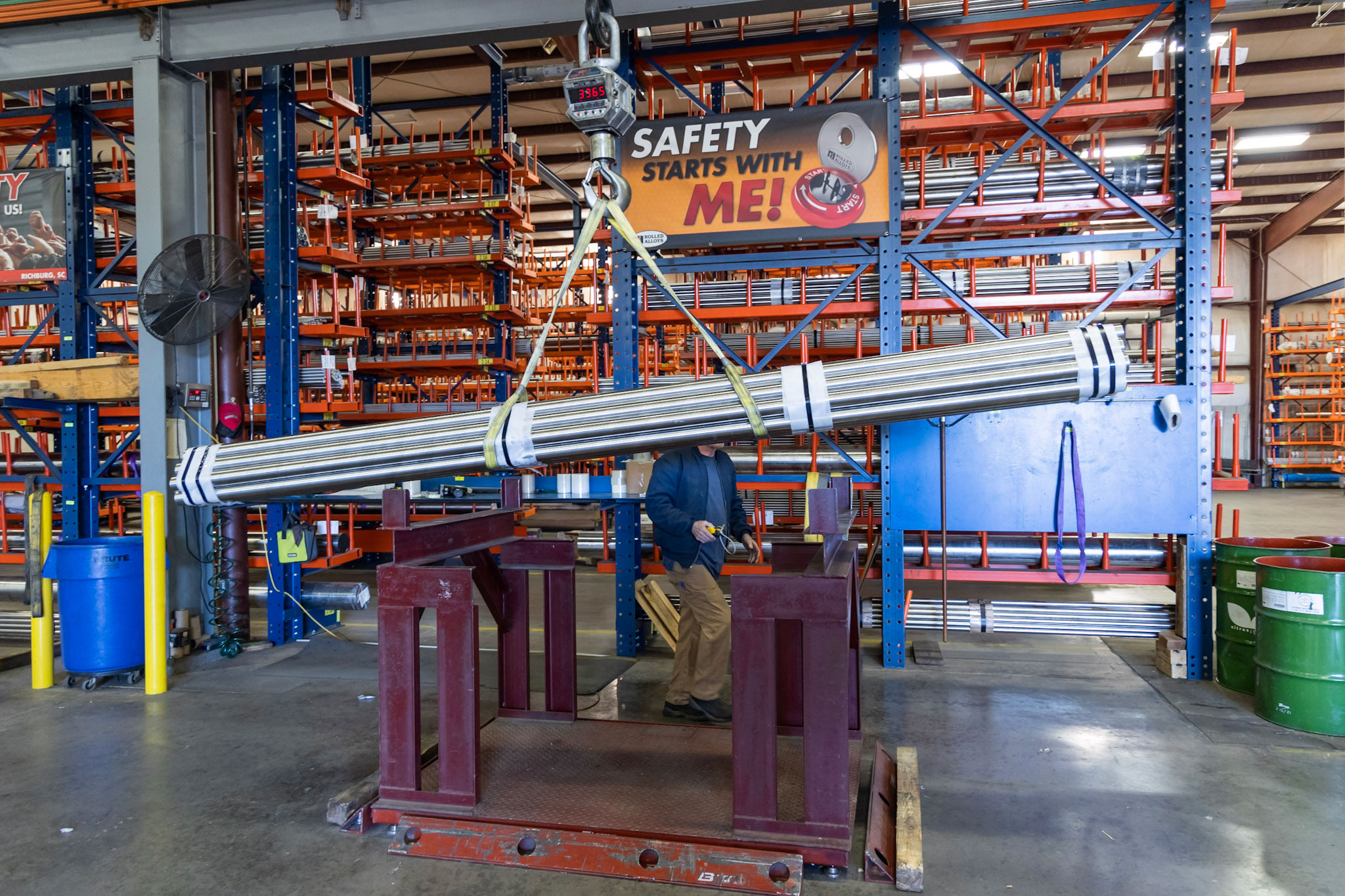
Manganese Substitution Grades of Stainless Steel
Explore the evolution of stainless steel with manganese substitution grades, offering cost-effective solutions for industries like appliances and food equipment.
Weld Wire Wizard
When dealing with specialty alloys, there can be unique welding combinations where guidance is not easily found. The Rolled Alloys Weld Wire Wizard helps to take guesswork out of the equation by allowing you to select any two alloys and see which weld wire is preferred. This tool was created with welders and fabricators in mind to determine the best wire options for their specific alloys or applications.
Filler per Lineal Foot w/ Reinforcement / Approximate Weight In Pounds (.lbs) | ||||
---|---|---|---|---|
Joint Design | Plate Thickness (.in) | GTAW / TIG | Electrodes Required (A) | GMAW Wire Required (B) |
Single Fillet | 1/8 3/16 1/4 3/18 1/2 5/8 |
0.032 0.072 0.13 0.29 0.52 0.80 |
0.064 0.144 0.26 0.58 1.03 1.61 |
0.038 0.085 0.015 0.34 0.60 0.94 |
"V" Groove | 1/4 3/8 1/2 |
0.37 0.62 |
0.73 1.23 1.7 |
0.43 0.73 1.00 |
Double "V" Groove | 1/2 5/8 3/4 1 |
0.77 0.95 1.32 1.83 |
1.53 1.90 2.63 3.65 |
0.90 1.12 1.55 2.16 |
Premium E-Grade
Specifications
Rolled Alloys supplies weld wire to meet requirements of AMS, AWS, and to aerospace companies such as Pratt & Whitney and GE. Most weld filler is covered by the American Welding Society [AWS] specifications. The Aerospace welding wires are predominately purchased to an AMS spec, whether or not they also have AWS.
Alloy Identification [LCS]
Flag Tagging
Flag tagging is a positive method of identification. The tag has a pressure sensitive adhesive backing and is placed approximately 1.2″ from the end of the rod. Tags are printed with alloy and specifications for identification. Custom tags are available.
Custom Packaging [AMS 2814]
Centerless Grinding
Spooled Wire
Wire Cleaning
Straightening and Cutting Capabilities
Length: From 12″ +/-0.010″ Lengths to 72″ Lengths
Types Of Welding
GMAW [Gas Metal Arc Welding]
GTAW [Gas Tungsten Arc Weld]
SMAW [Shielded Metal Arc Weld]
SAW [Submerged Arc Weld]
FCAW [Flux Cored Arc Welding]
Do you have a question about a weld wire selection?
Share This Page